Subscribe to Newsletter
Subscribe to Newsletter
Subscribe to Newsletter
Subscribe to Newsletter
Subscribe to Newsletter
Subscribe to Newsletter
Subscribe to Newsletter
Subscribe to Newsletter
Subscribe to Newsletter
Subscribe to Newsletter
Subscribe to Newsletter
Subscribe to Newsletter
Subscribe to Newsletter
Subscribe to Newsletter
Subscribe to Newsletter
Subscribe to Newsletter
Subscribe to Newsletter
Subscribe to Newsletter
Subscribe to Newsletter
Subscribe to Newsletter
Subscribe to Newsletter
Subscribe to Newsletter
Subscribe to Newsletter
Subscribe to Newsletter
Subscribe to Newsletter
Subscribe to Newsletter
Subscribe to Newsletter
Subscribe to Newsletter
Subscribe to Newsletter
Subscribe to Newsletter
Subscribe to Newsletter
Subscribe to Newsletter
Subscribe to Newsletter
Subscribe to Newsletter
Subscribe to Newsletter
Subscribe to Newsletter
Subscribe to Newsletter
Subscribe to Newsletter
Subscribe to Newsletter
Subscribe to Newsletter
Subscribe to Newsletter
Subscribe to Newsletter
Subscribe to Newsletter
Subscribe to Newsletter
Subscribe to Newsletter
Subscribe to Newsletter
Subscribe to Newsletter
Subscribe to Newsletter
Subscribe to Newsletter
Subscribe to Newsletter
Subscribe to Newsletter
Subscribe to Newsletter
Subscribe to Newsletter
Subscribe to Newsletter
Subscribe to Newsletter
Subscribe to Newsletter
Subscribe to Newsletter
Subscribe to Newsletter
Subscribe to Newsletter
Subscribe to Newsletter
Subscribe to Newsletter
Subscribe to Newsletter
Subscribe to Newsletter
Subscribe to Newsletter
Subscribe to Newsletter
Subscribe to Newsletter
Subscribe to Newsletter
Subscribe to Newsletter
Subscribe to Newsletter
Subscribe to Newsletter
Subscribe to Newsletter
Subscribe to Newsletter
Subscribe to Newsletter
Subscribe to Newsletter
Subscribe to Newsletter
Subscribe to Newsletter
Subscribe to Newsletter
Subscribe to Newsletter
Subscribe to Newsletter
Subscribe to Newsletter
Subscribe to Newsletter
Subscribe to Newsletter
Subscribe to Newsletter
Subscribe to Newsletter
Subscribe to Newsletter
Subscribe to Newsletter
Subscribe to Newsletter
Subscribe to Newsletter
Subscribe to Newsletter
Subscribe to Newsletter
Subscribe to Newsletter
Subscribe to Newsletter
Subscribe to Newsletter
Subscribe to Newsletter
Subscribe to Newsletter
Subscribe to Newsletter
Subscribe to Newsletter
Subscribe to Newsletter
Subscribe to Newsletter
Subscribe to Newsletter
Subscribe to Newsletter
Subscribe to Newsletter
Subscribe to Newsletter
Subscribe to Newsletter
Subscribe to Newsletter
Subscribe to Newsletter
Subscribe to Newsletter
Subscribe to Newsletter
Subscribe to Newsletter
Subscribe to Newsletter
Subscribe to Newsletter
Subscribe to Newsletter
Subscribe to Newsletter
Subscribe to Newsletter
Subscribe to Newsletter
Subscribe to Newsletter
Subscribe to Newsletter
Subscribe to Newsletter
Subscribe to Newsletter
Subscribe to Newsletter
Subscribe to Newsletter
Subscribe to Newsletter
Subscribe to Newsletter
Subscribe to Newsletter
Subscribe to Newsletter
Subscribe to Newsletter
Subscribe to Newsletter
Subscribe to Newsletter
Subscribe to Newsletter
Subscribe to Newsletter
Subscribe to Newsletter
Subscribe to Newsletter
Subscribe to Newsletter
Subscribe to Newsletter
Subscribe to Newsletter
Subscribe to Newsletter
Subscribe to Newsletter
Subscribe to Newsletter
Subscribe to Newsletter
Subscribe to Newsletter
Subscribe to Newsletter
Subscribe to Newsletter
Subscribe to Newsletter
Subscribe to Newsletter
Subscribe to Newsletter
Subscribe to Newsletter
Subscribe to Newsletter
Subscribe to Newsletter
Subscribe to Newsletter
Subscribe to Newsletter
Subscribe to Newsletter
Subscribe to Newsletter
Subscribe to Newsletter
Subscribe to Newsletter
Subscribe to Newsletter
Subscribe to Newsletter
Subscribe to Newsletter
Subscribe to Newsletter
Subscribe to Newsletter
Subscribe to Newsletter
Subscribe to Newsletter
Subscribe to Newsletter
Subscribe to Newsletter
Subscribe to Newsletter
Subscribe to Newsletter
Subscribe to Newsletter
Subscribe to Newsletter
Subscribe to Newsletter
Subscribe to Newsletter
Subscribe to Newsletter
Subscribe to Newsletter
Subscribe to Newsletter
Subscribe to Newsletter
Subscribe to Newsletter
Subscribe to Newsletter
Subscribe to Newsletter
Subscribe to Newsletter
Subscribe to Newsletter
Subscribe to Newsletter
Subscribe to Newsletter
Subscribe to Newsletter
Subscribe to Newsletter
Subscribe to Newsletter
Subscribe to Newsletter
Subscribe to Newsletter
Subscribe to Newsletter
Subscribe to Newsletter
Subscribe to Newsletter
Subscribe to Newsletter
Subscribe to Newsletter
Subscribe to Newsletter
Subscribe to Newsletter
Subscribe to Newsletter
Subscribe to Newsletter
Subscribe to Newsletter
Subscribe to Newsletter
Subscribe to Newsletter
Subscribe to Newsletter
Subscribe to Newsletter
Subscribe to Newsletter
Subscribe to Newsletter
Subscribe to Newsletter
Subscribe to Newsletter
Subscribe to Newsletter
Subscribe to Newsletter
Subscribe to Newsletter
Subscribe to Newsletter
Subscribe to Newsletter
Subscribe to Newsletter
Subscribe to Newsletter
Subscribe to Newsletter
Subscribe to Newsletter
Subscribe to Newsletter
Subscribe to Newsletter
Subscribe to Newsletter
Subscribe to Newsletter
Subscribe to Newsletter
Subscribe to Newsletter
Subscribe to Newsletter
Subscribe to Newsletter
Subscribe to Newsletter
Subscribe to Newsletter
Subscribe to Newsletter
Subscribe to Newsletter
Subscribe to Newsletter
Subscribe to Newsletter
Subscribe to Newsletter
Subscribe to Newsletter
Subscribe to Newsletter
Subscribe to Newsletter
Subscribe to Newsletter
Subscribe to Newsletter
Subscribe to Newsletter
Subscribe to Newsletter
Subscribe to Newsletter
Subscribe to Newsletter
Subscribe to Newsletter
Subscribe to Newsletter
Subscribe to Newsletter
Subscribe to Newsletter
Subscribe to Newsletter
Subscribe to Newsletter
Subscribe to Newsletter
Subscribe to Newsletter
Subscribe to Newsletter
Subscribe to Newsletter
Subscribe to Newsletter
Subscribe to Newsletter
Subscribe to Newsletter
Subscribe to Newsletter
Subscribe to Newsletter
Subscribe to Newsletter
Subscribe to Newsletter
Subscribe to Newsletter
Subscribe to Newsletter
Subscribe to Newsletter
Subscribe to Newsletter
Subscribe to Newsletter
Subscribe to Newsletter
Subscribe to Newsletter
Subscribe to Newsletter
Subscribe to Newsletter
Subscribe to Newsletter
Subscribe to Newsletter
Subscribe to Newsletter
Subscribe to Newsletter
Subscribe to Newsletter
Subscribe to Newsletter
Green Hydrogen: Powering Industries Towards Net-Zero Emissions
Fueling the Future with Green Energy Innovations
Enroll now for early access of e-LMS
Online/ e-LMS
Self Paced
Moderate
1 Month
About
The Green Hydrogen Program is a dynamic and interactive event designed to delve into the potential of green hydrogen as a game-changer in decarbonizing industries. Join us for a day filled with insightful presentations, engaging discussions. Discover the latest advancements, technologies, and best practices in leveraging green hydrogen to drive sustainable transformations across various industrial sectors. The program on the use of green hydrogen in fuel cell electric vehicles and its potential to replace fossil fuels is a gathering of experts, researchers, and professionals in the field of alternative energy, sustainable transportation, and hydrogen fuel cells. Collaborate with like-minded professionals, exchange ideas, and uncover innovative solutions to accelerate the adoption of green hydrogen on a global scale.
Aim
The aim of this program would be to provide participants with a comprehensive understanding of green hydrogen, its production, and its potential applications in industry. The program would cover the basics of green hydrogen, its benefits over traditional hydrogen production methods, and its potential for reducing greenhouse gas emissions.The program will explore the potential of green hydrogen as a clean and renewable energy source for powering fuel cell electric vehicles. It will cover topics such as the production, storage, and distribution of green hydrogen, its use in fuel cell electric vehicles, and its potential to replace fossil fuels.
Program Objectives
- Understand the fundamentals and importance of green hydrogen in achieving net-zero emissions.
- Explore the technological methods of producing and storing hydrogen energy.
- Analyze the economic viability and environmental impact of hydrogen energy solutions.
- Study the regulatory and policy environment affecting green hydrogen adoption.
- Develop project management skills specific to renewable energy projects.
Program Structure
Module 1: Introduction to Green Hydrogen and its Applications
- Introduction to hydrogen and its applications in industry
- Overview of different applications of green hydrogen in industry, such as transportation, energy storage, and industrial processes
- Comparison of traditional hydrogen production methods with green hydrogen production
- Basics of electrolysis and how it produces green hydrogen
- Understanding the benefits of green hydrogen and its potential to reduce greenhouse gas emissions
Module 2: Green Hydrogen Production and Infrastructure
- Overview of green hydrogen production technologies and their advantages and disadvantages
- Selection of suitable renewable energy sources for green hydrogen production
- Understanding the process of electrolysis and different types of electrolysers
- Design and construction of a green hydrogen production system
- Infrastructure and storage of green hydrogen
Module 3: Materials in Green Hydrogen Production
- Characterizing a material for electrocatalytic green hydrogen production.
- 2D Materials (M-Xenes) in Green Hydrogen Production and Storage
- Characterizing Materials for Photo-electrochemical Green Hydrogen Production
- GoI Policies on Green Hydrogen.
Note:
- On Day 2
Participants can learn about the electrolyzers, their assembly and working, and different types.
- On Day 3
Participants will get real time examples on electrocatalyst characterization for electrolyzers and will learn ways to characterize the materials for hydrogen.
Module 4: Green Hydrogen in the transportation Sector
- What is Green Hydrogen and its properties?
- The benefits and challenges of using Green Hydrogen as a fuel
- The potential of Green Hydrogen to replace fossil fuels in the transportation sector
- Understanding the different types of fuel cell electric vehicles
- Overview of their components and working
- Different methods of Green Hydrogen production and their suitability for fuel cell electric vehicles
- Electrolysis process and types
- Photovoltaic and wind energy methods
- Biomass conversion methods
Module 5:Fuel Cell Technology & Electric Vehicle Components
- Understanding of fuel cell technology and its functioning
- Components of fuel cell and their working
- Types of fuel cells and their application in vehicles
- Efficiency and limitations of fuel cells
- Understanding the components of fuel cell electric vehicles
- Types of electric motors and batteries used in fuel cell electric vehicles
- Working and integration of fuel cell, electric motor, and battery
- Factors affecting the performance and efficiency of fuel cell electric vehicles
- Range, acceleration, and speed analysis of fuel cell electric vehicles
- Comparison of fuel cell electric vehicles with traditional vehicles
Module 6:Establishing Hydrogen Fueling Infrastructure,Policy and Regulations
- The challenges and opportunities in establishing hydrogen fueling infrastructure in India
- Types of hydrogen fueling stations and their suitability for Indian conditions
- Cost and feasibility analysis of establishing hydrogen fueling infrastructure
- The role of policy and regulations in promoting Green Hydrogen adoption
- National and international policies promoting Green Hydrogen adoption
- Regulatory framework for Green Hydrogen production, transportation, and storage
- The cost analysis of Green Hydrogen production and its application in fuel cell electric vehicles
- The potential of Green Hydrogen to reduce carbon emissions and achieve climate goals
- Carbon footprint analysis of Green Hydrogen-based transportation
Participant’s Eligibility
- Undergraduate degree in Environmental Science, Chemical Engineering, or related fields.
- Professionals in the energy sector or agricultural industries.
- Individuals with a keen interest in renewable energy and sustainable practices.
Program Outcomes
- Mastery of green hydrogen technologies and applications
- Understanding of the environmental benefits of hydrogen energy
- Skills in economic analysis of energy projects
- Knowledge of global energy policies
- Ability to design and implement hydrogen-based energy solutions
Fee Structure
Standard Fee: INR 4,998 USD 110
Discounted Fee: INR 2499 USD 55
We are excited to announce that we now accept payments in over 20 global currencies, in addition to USD. Check out our list to see if your preferred currency is supported. Enjoy the convenience and flexibility of paying in your local currency!
List of CurrenciesBatches
Live
Certificate
Program Assessment
Certification to this program will be based on the evaluation of following assignment (s)/ examinations:
Exam | Weightage |
---|---|
Mid Term Assignments | 50 % |
Project Report Submission (Includes Mandatory Paper Publication) | 50 % |
To study the printed/online course material, submit and clear, the mid term assignments, project work/research study (in completion of project work/research study, a final report must be submitted) and the online examination, you are allotted a 1-month period. You will be awarded a certificate, only after successful completion/ and clearance of all the aforesaid assignment(s) and examinations.
Program Deliverables
- Access to e-LMS
- Real Time Project for Dissertation
- Project Guidance
- Paper Publication Opportunity
- Self Assessment
- Final Examination
- e-Certification
- e-Marksheet
Future Career Prospects
- Green Hydrogen Specialist
- Renewable Energy Consultant
- Energy Transition Strategist
- Sustainability Officer
- Policy Advisor on Energy Sector
- Research Scientist in Energy Technologies
Current Participants* Analytics
Country
Profession
Affiliation
Note: The information shown in the above-mentioned analytics is live and may include information that is not completely correct like spelling mistakes, grammatical mistakes , factual errors or even mis representation as this is what participants have entered, the information is currently not edited and or filtered , but at later stages they will be filtered to provide true data representation.
Enter the Hall of Fame!
Take your research to the next level!
Achieve excellence and solidify your reputation among the elite!
Related Courses
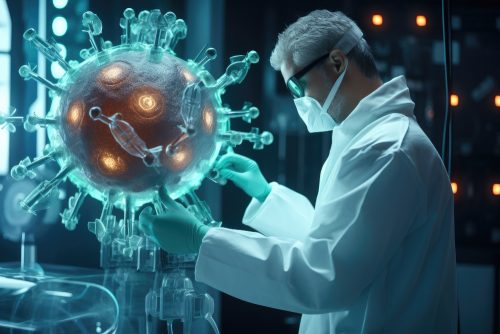
Artificial Intelligence for …

AI Applications in Pharmacy: …
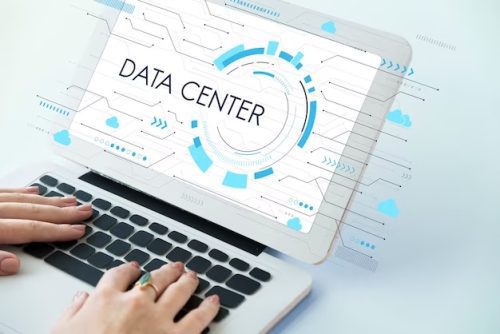
SQL & Power BI Program: …
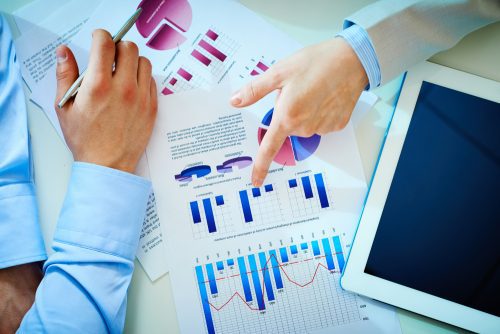
Data Visualization using …
Recent Feedbacks In Other Workshops
The course was more theoretical than practical.
nothing